Tidal Power
Tidal barrages have been used to generate power for decades, but environmental and economic issues have prevented their widespread adoption. Much more promising are tidal stream technologies - essentially underwater wind turbines. Though only in the early stages of development, they have the potential to be a large-scale energy source in future.
Tidal Power
By the Numbers
15 metres
Tidal range of Nova Scotia's Bay of Fundy, the largest in the world
152,000 MW
Total potential capacity of the 27 best locations in the world for tidal stream farms
3.7 cents/kWh
Cost of power generated by the La Rance in France tidal barrage, cheaper than most competitors
15,900 MW
Generating capacity of the proposed Severn tidal lagoons in the UK, costing $65 billion
$0.54 to $0.66 per MW
Cost of electricity from the first tidal stream farms, comparable to the cost of wind power in 1980
19
Countries currently investing in tidal power research
Last Updated: February 2017
Andrew Farris and Charlotte Helston
Tidal power exploits energy drawn from the movement of ocean tides to produce electricity. There are two main branches of technology that tap the tides for energy. The first and best known are tidal range technologies which harness power through dam-like structures that trap rising waters on one side and then release it back to the other through turbines that spin to generate electricity. The second technology is newer and just beginning to be tested out on a commercial scale around the world, so-called tidal stream technologies. They harness fast-flowing currents to spin turbines. Tidal stream turbines come in many shapes and sizes but the most common designs are basically underwater wind turbines.
Tidal energy is considered renewable because the tides move on a predictable, daily schedule, depending only on the orbits of the Earth, Moon, and Sun, and are essentially inexhaustible. Though tidal energy is carbon free, tidal range technologies have not proven to be environmentally benign. Concerns over the health of shoreline and aquatic ecosystems mar this otherwise clean source of energy.
Until the last decade large-scale tidal range systems dominated the tidal power scene. Yet severe environmental and economic drawbacks to this technology quickly became evident and this has stymied its development and prevented it from taking off. Though it has been around since the 1960s only a handful of tidal barrages have been built around the world.
The focus of research and development has shifted from tidal range systems to tidal stream technologies. Though more environmental studies have to be conducted on these new technologies, at this time they appear very promising. Power from tidal stream turbines is still very expensive, but the technology is still in its infancy and the industry today is often likened to the wind power industry 20 years ago. At that time wind power was hugely expensive but continued investment succeeded in bringing the price of wind farms down to where they are today, economically competitive with practically every power source.
Within Canada the provinces of Nova Scotia and BC are fantastically well suited for tidal development, given their tidal geography, and Nova Scotia is investing heavily in a future tidal power industry, and so far those investments appear to be beginning to pay off as foreign companies are setting up shop in the province to test out their tidal power designs. British Columbia got an early lead in tidal stream development with the Race Rocks Tidal Project in 2006, but has since fallen behind and the once promising industry is struggling to survive. Below we talk about
How Tidal Power Works
- There are two main tidal power technologies called tidal range and tidal stream.
- Tidal range facilities act like dams that trap the tides and then release the water to generate electricity.
- Tidal stream technologies can be thought of as similar to underwater wind turbines.
Tides are created by the gravitational pull of the sun, moon and the rotation of the earth and tidal power generators work by harnessing their natural ebb and flow. Tidal energy can be harnessed both in the sea, and in tidal rivers and estuaries. The ebb and flow of tides can occur once or twice a day depending on location. Due to the upward gravitational rotation of the moon, the water level rises gradually until it reaches its highest point and then gradually falls back to its lowest point. One of the main advantages of tidal power over solar or wind power is that the tides are entirely predictable and power can be scheduled years in advance. The tide does not occur at the same time every day but rather fluctuates over a period of roughly two weeks.
There are two main tidal power generation technologies. The first are tidal range technologies that rely on the rise and fall of sea level to generate power. The second, tidal stream technologies, harness the currents created by tides.

BBC
The most common form of tidal range technology is the tidal barrage. It is essentially an adaptation of conventional hydroelectric dam technology. A wall is built that blocks off an existing tidal estuary with a dam, or barrage. Movable flood gates on the barrage—called sluice gates—allow incoming tidal waters to fill up in a reservoir. Once the water reaches its maximum level, the gates close and trap the water. This trapped water is called hydrostatic head.
As the tide ebbs, a gradually increasing head differential is created between receding water levels and the fixed level within the barrier. When the head differential has reached the desired value, the potential energy created can be converted into mechanical energy and then electrical energy by allowing the water to flow out through turbines. A proper site for this type of technology should have sufficient tidal range and the best locations are in natural bays. It is also important to locate the facility in such a way that it will not dramatically reduce the tidal range. One major drawback of tidal barrages is that the tide only goes out for so much time per day, and power is generated for as little as four hours a day, giving barrages low levels of efficiency in the 20-25% range.
Tidal barrage technology is not new and mills that use the tide for power date back to the 8th Century CE. The tidal mills were mainly used for grain grinding and were of similar design to the conventional water mills with the addition of a dam and reservoir. The technology fell out of favour after the Industrial Revolution until the 1960s to 1980s when experimental tidal barrages were built in France, Russia, China and Nova Scotia.
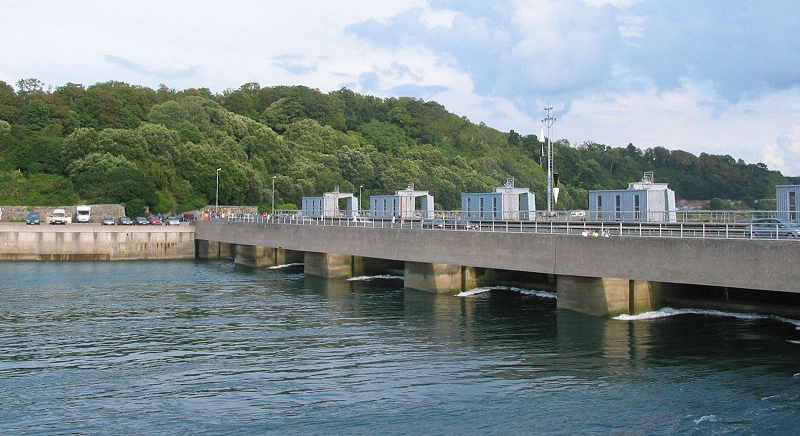
Wikipedia
Tidal Lagoons and Dynamic Tidal Power
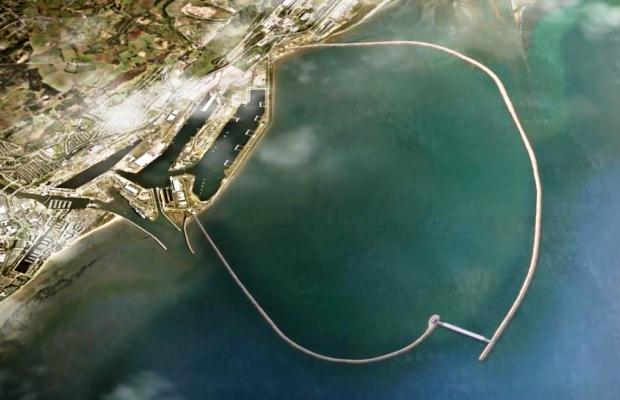
4coffshore
Past experience with tidal barrages has shown that walling off fragile and ecologically important coastal ecosystems, even for only a few hours a day, can be environmentally damaging, something we discuss in more detail below. As a result scientists and engineers have proposed several novel tidal range alternatives to the barrage, the furthest advanced being the tidal lagoon. Tidal lagoons would not be attached to the shoreline at all, but rather be artificially created pools in the sea itself that would let water in and out and generate power much the same way as tidal barrages, except with greater efficiency and without isolating ecologically sensitive inter-tidal areas.
As of early 2016 the first tidal lagoon project is under construction off the coast of the Welsh city of Swansea, enclosing around 11 km2 of water. It will produce 320 MW of power for 14 hours a day, enough to power 155,000 homes and making it the largest tidal energy facility in the world. Scheduled for completion in 2019, if successful it will be the first of six proposed tidal lagoon projects to be built on Britain’s west coast.
An even more radical and promising tidal range proposal is the Dutch-designed Dynamic Tidal Power system. A giant T-shaped pier would be built up to 60 km straight out from the coast, blocking tides that move parallel to the coast and cause enough head differential to could produce tremendous amounts of electricity, while possibly avoiding many of the economic and environmental problems of other tidal range technologies. No such projects have been built yet, but teams from China and the Netherlands are moving forward with planning on such projects.
Tidal Stream Technologies
Tidal stream technologies are the second class of tidal power generation schemes, and they act much like underwater wind turbines, generating power from the kinetic energy of fast-flowing tidal currents. The generators are sunk 20-30 meters, and can be situated anywhere that possesses a strong tidal flow.
Tidal stream technologies have seen huge advances in the last decade, with many companies around the world working to recreate the success of wind turbines on land in the underwater realm. Yet developing a successful tidal current turbine is much more difficult than simply dropping a wind turbine in the ocean.
Because water is about 800 times denser than air tidal stream turbines must be built much sturdier than their terrestrial counterparts, though they can also spin much more slowly, around 7-11 rotations per minute. Shrunken diameters help to reduce the structural strain. The advantage of greater density of water is that relatively large amounts of power can be produced with relatively small rotor diameters. For example: rotors with a diameter of 10-15 meters can generate as much as 700 kW of power, whereas a 600 kW wind turbine requires a rotor diameter of 45 meters. Tidal turbines function best at flow rates of 7-11 km/hr. An irrefutable advantage of tidal turbines over wind turbines is their predictability: Tides flow in and out every day, promising daily, schedulable energy.
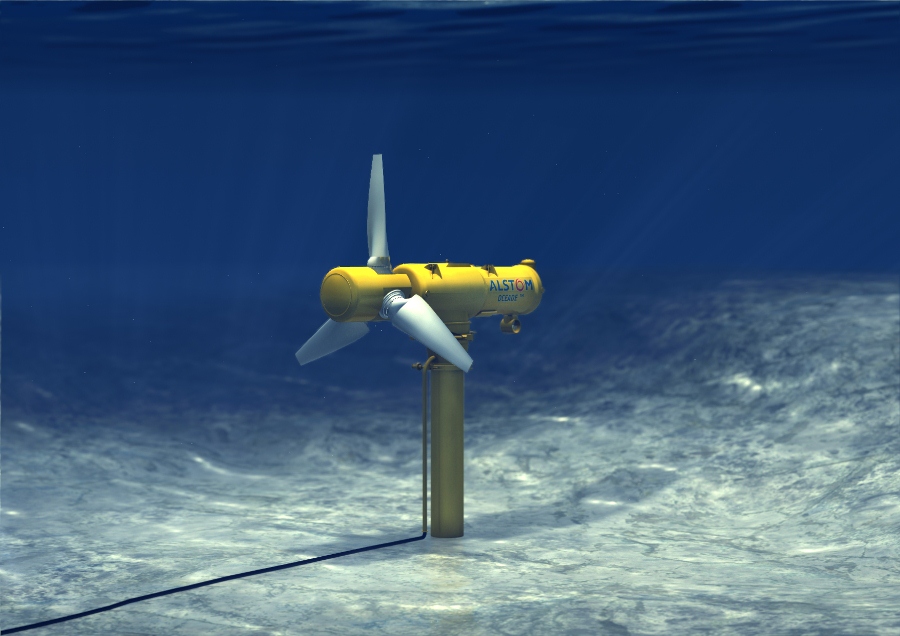
Subseaworldnews
Yet the technology is far less mature than wind power, and tidal stream technologies are just now leaving the prototype and demonstration phase. Indeed, no single design of tidal turbine has been agreed upon yet. Companies around the world are pioneering 40 different designs, and while most function on the same principles as horizontal axis wind turbines, there are a host of other more novel designs such as vertical-axis turbines, rotating screws, tidal kites, and paddlewheels.
Research still needs to be done in a number of fields, the most important of which is making the turbines durable enough to survive the hostile aquatic environment: A test turbine in Nova Scotia had its rotors ripped off by the immense tidal forces in the Bay of Fundy. Corrosive salt water also takes a serious toll on equipment. They also need to be made more efficient and economical as the power they produce today remains prohibitively expensive. Experiments are also ongoing on the best method for mooring the turbines to the seafloor. While most common are those put on concrete bases on the sea bed, some designs have been mounted on towers, or are even made to float in the water, tethered to the seafloor with cables. Research is also being conducted on how best to hook these turbines up to the grid.
Ultimately, as the technology advances and the price comes down, as it inevitably will with continued investment, farms of tidal stream turbines will be laid on the seafloor (or floated above it). One field of ongoing research is determining the proper spacing and siting of the turbines to maximize power output. Avoiding this altogether is building tidal fences, a concrete structure filled with tidal turbines placed in a region of fast-flowing water, a channel between two land masses for instance. Fence installations are presumed to be less expensive to develop than tidal barrages, as well as less harmful to marine ecosystems.
No tidal fences, or farms of tidal current turbines, are yet in operation, though the first farm is currently under construction in Scotland. Their main component after all — tidal current turbines — are still in the development stages. But the technology is rapidly maturing and appears on the verge of a tipping point, much as wind and solar were 20 years ago.
Great leaps in the technologies are already being envisioned. All the designs under discussion right now are first generation tidal turbines and cannot operate deeper than 30 metres. Second generation devices, which may start entering the demonstration phase by 2020, will be much larger, generate much more power, and can be placed at much greater depths, opening up great new swaths of the ocean to tidal development.
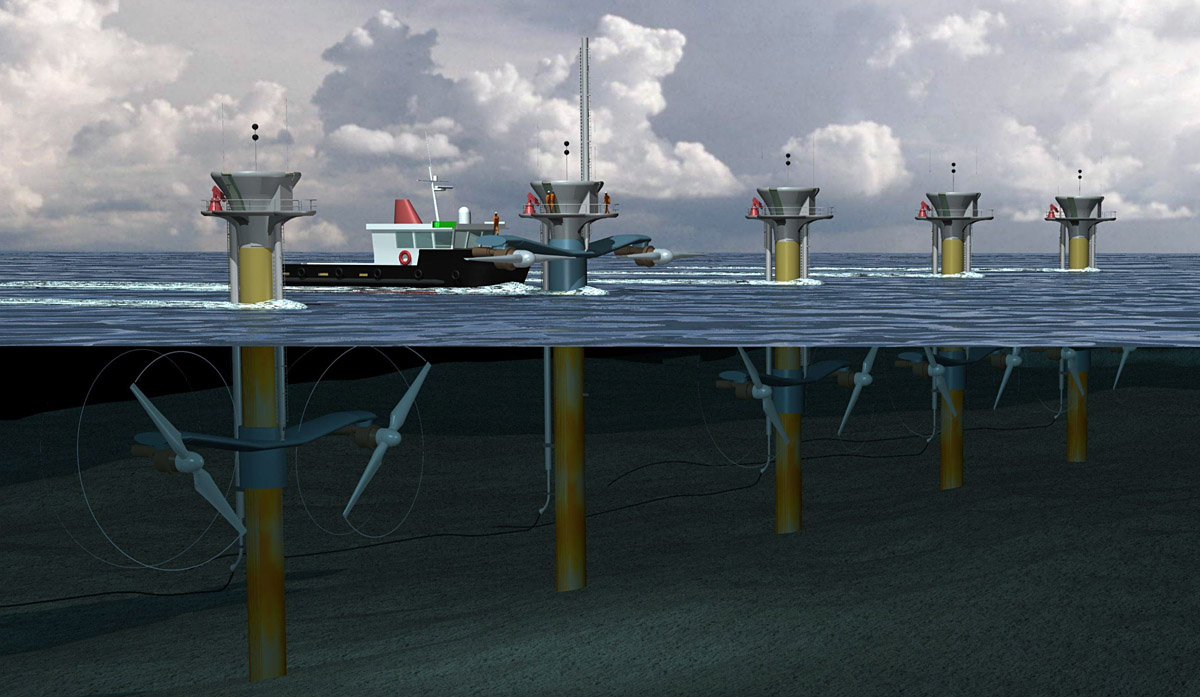
Marine Turbines
- Ocean Energy Council, "What is Tidal Energy."
- Charlier. 2003.
- Hammons, 1993.
- Tidal Lagoon Power, "Swansea Bay."
- Renewable Energy World, "Advances in Dynamic Tidal Power Technology."
- Harvey, 2010.
- Aubrecht, 2006.
- Garrett & Cummins, 2005.
Geography of Tidal Power
- Tidal barrages are limited to locations like bays and estuaries.
- There are many more locations where tidal stream turbines can be placed so long as the currents are fast enough.

Samuel Wantman
Tidal power technology is only useful if it is employed in favourable conditions. Location is everything. For tidal barrages a tidal range of at least 7 metres is required, while tidal turbines need tidal currents moving at speeds of 7-11 km/hr. In addition both types must have stable conditions for a barrier or turbine to be built into. Often, good sites are located in areas where incoming waters are funneled into narrow channels, bays, river mouths and fjords.
There are only a limited number of places around the world where the tidal range is great enough to justify a barrage. The world's greatest tidal range is found in Canada's Bay of Fundy, where it is over 15 meters. Quebec’s Ungava Bay and numerous estuaries around the Pacific Northwest feature ideal tidal ranges as well. Around the world sites on the coasts of Argentina, Australia, India, South Korea, Mexico, the US and Russia offer the best potential sites for tidal barrage plants.
Developers of tidal turbines are seeking out locations that possess tidal streams — areas of quickly flowing water caused by the motion of the tides. Typically, tidal streams are found where underwater valleys force currents to constrict and speed up. These are generally more common and located nearer economic centres where the power would be useful.
Major tidal currents occur in the Bay of Fundy, the Gulfs of Saint Lawrence and Mexico, the Amazon and Rio de la Plata river estuaries, and straits like the Straits of Magellan, Gibraltar, Sicily, the Skagerrak-Kattegat separating Denmark and Sweden, and the Bosporus in Turkey. In the Far East, useful currents are found near Taiwan, Korea, and the Kurile Islands north of Japan.
The United Kingdom, whose rugged coast is punctuated by inlets and surrounded by islands, is extremely well suited for both kinds of tidal development and has taken an early lead in the field. These locations include the Bristol Channel, Pentland Firth, the Hebrides, in the Irish Sea, the North Channel, Alderney Race, the Isle of Wight, the Orkneys and the Shetlands. A recent study by Oxford University found Scotland’s Pentland Firth to be “almost certainly the best site for tidal stream power in the world,” with enough potential energy to fulfill half of Scotland’s power needs.
How much power could this all add up to? There are considerable quantities of untapped tidal energy in waters all around the world and it is difficult to precisely calculate just how much power could be derived from it. One estimate by the International Renewable Energy Agency puts global potential resources at 3 TW. A study by the European Innovation Partnerships for Water, a think tank, picked the 27 most promising locations for tidal barrages in the world and pegged their total capacity at 152,000 MW. Tidal lagoons and Dynamic Tidal Power plants could theoretically multiply that number several times over.
The total potential of tidal stream technologies will likely change as the technology matures and more countries begin carefully mapping their tidal resources, a task the leading countries only began recently. Nevertheless, Atlantis Resources, a British Tidal power company, estimates 90,000 MW of generating capacity could be built, for a total of 150 TWh of power a year.
=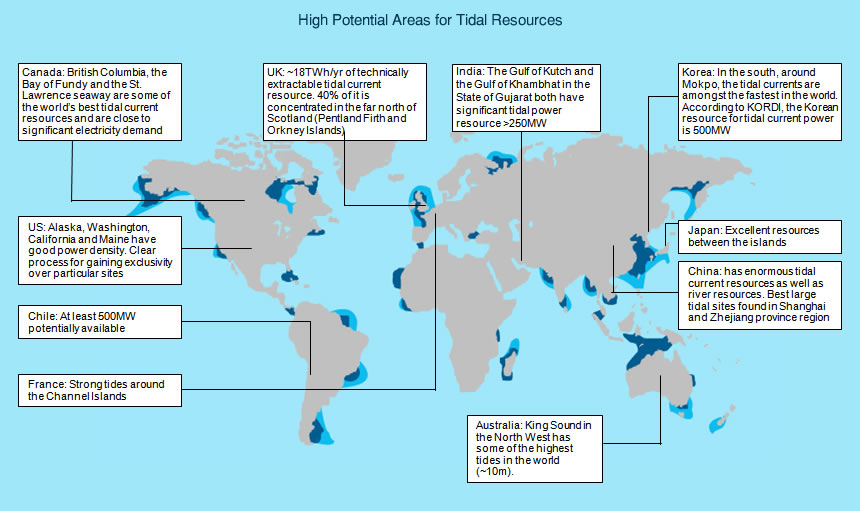
Wikispaces
- Colazingari, 2008.
- Aubrecht, 2006.
- Charlier. 2003.
- Tidal Energy Today, "Estimate of global potential tidal resources."
- International Renewable Energy Agency, 2014.
- European Innovations Projects, “Annex 6 Potentials for tidal barrages, tidal flows and osmotic power.”
- European Innovations Projects, “Annex 6 Potentials for tidal barrages, tidal flows and osmotic power.”
Economics of Tidal Power
- Tidal power is currently very expensive but the price of tidal stream turbines may drop sharply as the technology develops.
The Economics of Tidal Range Technologies
Large tidal barrages present three main problems for skittish investors: they have large up-front capital costs, long construction times and produce relatively limited quantities of power. This is somewhat balanced out by operational lives of 100 years. Once over that initial capital building cost hump, tidal barrages become attractive investments. Studies peg maintenance and operations over the barrage’s century long lifespan at less than 0.5% of initial capital costs. Much depends on the site, the two decisive economic inputs being the size of the reservoir basin and the tidal range. The first determines how long power can be produced and the second at what efficiency. Costs generally increase for sites that experience violent winds and waves, as dykes must be built stronger and larger to withstand them.
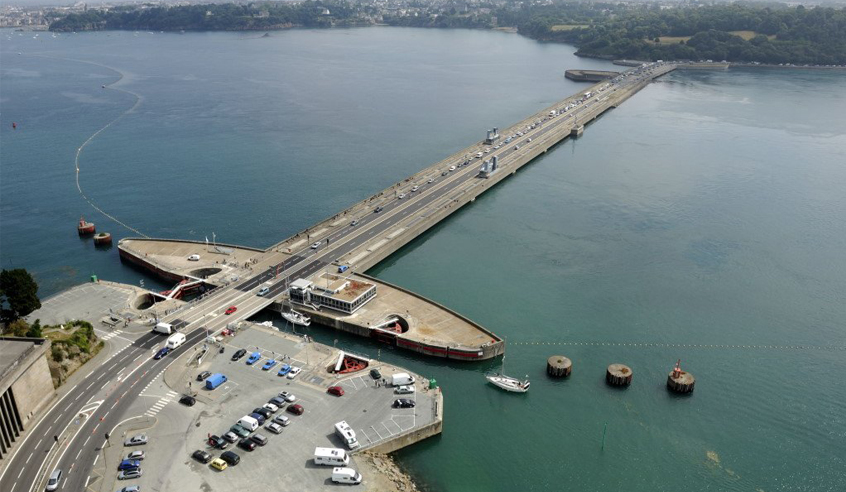
EDF Pulse
Despite having been around for decades, tidal barrage technology is not well developed. There are only four real examples to draw economic conclusions from, not enough to have any clear idea of just how expensive any proposed barrage will end up being. An estimate is given by researcher Eleanor Denny. She argued that in order for a tidal barrage facility to be profitable, its capital cost should be less $800,000 per MegaWatt of installed capacity. Unfortunately this is an unrealistically low cost. According to IRENA the La Rance project hurtled past this when their construction budget came out to $817 million in today’s dollars, or $3.4 million per MW. Korea’s Sihwa Lake design was much more economical costing $298 million, or $1.17 million a MW. Both barrages also double up as highway bridges which adds as much as 40% more value to the structure over and above mere power production. Nevertheless it appears Sihwa Lake was a model of economic stewardship that will be difficult to repeat, and few of the other proposed tidal barrage projects around the world even come close to Denny’s profitability target. This will likely be a significant roadblock to their eventual construction.
Once people get past the initial sticker shock however they find electricity prices to be a pleasant surprise. After 50 years of operation the La Rance power plant provides electricity at bargain-basement price of 3.7 cents/kWh, much cheaper than the 10.8 cents/kWh charged by coal thermal plants in the area. The cost is even lower than that of France's nuclear power, which is 3.8 cents/kWh. Only hydroelectric plants, at 3.2 cents, are more efficient. The price could be brought down much further if bi-directional turbines that generate electricity on both the ebb and flow of the tide, which will likely happen on future projects.
Tidal Lagoon Power is pushing a tremendously ambitious proposal for a series of six tidal lagoons off Britain’s western coast to be completed by 2027, each one bigger than the last for a combined total of 15,900 MW of generating capacity. Building these six facilities will cost some $65 billion, and in the process nurture a British tidal lagoon industry that can export their expertise around the world (Canada is at the very top of their list). Unfortunately the costs will be high, at $4 million per MW. On the other hand the economies of scale provided by this gigantic plan and their 120-year-lifespan would be huge advantages. A levelized cost analysis by Poyry, an engineering consultancy, found the tidal lagoons would be cost competitive with wind and solar power, and even nuclear and gas power which are the cheapest power sources available.
The Economics of Tidal Stream Technologies
Tidal stream technologies are essentially a decade old, and have only begun making great strides in the past five years. As yet the price is very high but it is almost certain dramatic price drops will accrue over time. Furthermore any scale of tidal stream turbines can be built as opposed to one giant project for barrages, giving developers much greater flexibility. Farms don’t have to be built in one go, like tidal barrages, but gradually expanded over time. An in-depth economic analysis by the Carbontrust found the bulk of costs in early tidal stream farms will be in the installation, about 35% of the total, with operations and maintenance (20%), station keeping (20%), power takeoff (10%), the structure (10%) and grid connection (5%) accounting for the remainder. Early British farms of about 10 MW will produce power at a range of $0.54 to $0.66 per KWh. This may sound expensive but we must remember this is roughly the cost of wind power in 1980.
Nevertheless these numbers leave much to be desired, especially as the 2012 Carbontrust estimate was actually double their previous 2006 estimate. The 2006 estimate was made before anyone had actually experimented with a tidal stream turbine in real world conditions, and only through experimentation did developers learn the huge technical challenges getting the technology to work posed. They also admitted that in 2006 their assessment of future decreases in price was over-optimistic about, a reminder that though it is likely tidal will one day be cheap enough to compete with other forms of renewable energy, it will take time.
Picking the best tidal sites is absolutely key to determining future energy cost. The two main determining factors for tidal stream projects are depth (the shallower the project the easier to install and maintain) and speed of tidal current (how much power can be had). If the best sites are developed early this will help bring costs down through learning, and allow a smoother rise in capacity. Building those early farms bigger is better too. The cost per MW of power in a 150 MW farm would likely be half that in a 10 MW farm. Finally as the tidal farm developers move along the learning curve they will discover cheaper and more efficient ways to build, install and repair every part of the turbine, from carbon-fibre rotor blades, lightweight fibre mooring systems and linear generators. All together costs will need to come down 50% to make tidal stream generation with offshore wind generation, itself on the expensive side of the spectrum of commercially viable renewables. This appears entirely achievable though how long it takes will depend entirely on the amount of money invested in pushing the limits of the technology.
Economic Effects on Tourism and Fishing
An increase in tourism has been observed at Canada's Annapolis tidal plant, as well as at France's La Rance plant. More than 40,000 tourists visit the Annapolis facility each year. Sites have a potential to double as information centers, employing individuals in a range of tourism positions, in addition to the general operation jobs created by the power plant itself. Temporary construction jobs are opened up as well during the installation of the facilities.
On the other hand negative environmental effects on marine life can be detrimental to the fishing industry. Some fishermen have raised concerns over the fact that most identified sites for tidal power are also key migration routes for fish. Additionally, sedimentation caused by tidal barrages could kill clams, while also damaging local shellfish fisheries. The La Rance facility displayed no major effects on the immediate fish community or local fisheries. The area, however, had a minute fishing industry to begin with and no professional fisherman after 1960. Impacts are expected to be much more apparent in locations where fish are abundant and fish passage is repeated by the same populations multiple times over the year, such as Canada's Bay of Fundy site.
- Hammons, 1993.
- Meygen Limited, “Pentland Firth/Inner Sound.”
- Meygen Limited, “Pentland Firth/Inner Sound.”
- Denny, 2010.
- Williams, 2010.
- Tidal Lagoon Power, "Swansea Bay."
- Poyry, 2014.
- Denny, 2010.
- Government of Denmark, 2015.
- Carbon Trust, 2011.
- European Innovations Projects, “Annex 6 Potentials for tidal barrages, tidal flows and osmotic power.”
- Johnson, 2006.
- American Fisheries Society, 2010.
- Pollack, 2008.
Tidal Power's Environmental Issues
- Tidal barrages can have large negative impacts on marine ecosystems, but lagoons may be better.
- Early research indicates tidal stream turbines do much less damage.
Environmental Effects of Tidal Barrages
The environmental effects of tidal barrages vary enormously from site to site, but they tend to be quite striking. A 2010 study examined ecological impacts at the Kislaya Guba tidal power plant in Russia. The 400 kW plant was completed in 1968 and continues to run to this day. An environmental evaluation of the Kislaya site sponsored by UNESCO provides a general assessment of the potential risks associated with tidal barrage power plants.
Prior to development, Kislaya Guba Bay was a fjord with a rich array of marine life. During the four years it took to construct the power plant, the bay was closed off from the sea by a dike. Water exchange was massively reduced to several percent of the natural exchange. The lack of moving water permitted the entire bay to freeze over in the winter, which annihilated coastal biota to a depth of 5m (15m where oxygen was depleted and accumulated hydrogen sulfide contaminated the water). Evidence of ecosystem damage can be found in the abundance of dead mollusks in the bay. The study did indicate some environmental recovery about 20 years after the initial construction, though it is not the same ecosystem it once was and continuing impacts of operation include: "diminution of tides, diminution of sea swells, reduction in the flow of fresh water from the partitioned water area to the sea, and the mechanical effect of the turbine on plankton and fish.” It is thought that with experience and better environmental assessments, future projects could avoid at least some of the pitfalls encountered by this Soviet-era engineering project.

In general, tidal barrages reduce the tidal range by about half; diminishing the intertidal zone and instigating a ripple of effects through the coastal ecosystem. The intertidal area provides a key feeding ground for birds. When the condition of this area is compromised, birds are likely to starve, or else forage for food in new ecosystems, potentially offsetting the natural balance there. The trapping of salt waters, where they would naturally flow into delicate salt marshes, can cause these areas to become diluted with fresh water, destroying a formerly intact ecosystem. Some estuaries may have formerly provided nurseries for breeding fish that would be jeopardized by tidal power development. It is also possible for fish and marine mammals to suffer damage or death by collision with the barrage or turbines, though fish passages and ladders can be used with varying degrees of success.
The macrotidal estuaries of the Bay of Fundy, for instance, are used by large numbers of migratory fish, including dogfish, sturgeon, herring, shad, Atlantic salmon and striped bass, as well as larger marine animals such as squid, sharks, seals and whales. Studies have shown that fish passage utilizing the Annapolis estuary has a disastrous turbine related mortality of 20-80% per passage depending on fish species. Injury or mortality of fish can occur in several ways during turbine passage, including mechanical strike, shear (the fish is caught between two streams with different velocities), pressure changes and cavitation (implosion of air bubbles which produces shock waves). The study of Annapolis estuary concluded "that introduction of tidal turbines into open ocean current systems will cause widespread impact on marine populations resulting in significant declines in abundance."
These environmental issues have made governments outside of Korea reluctant to invest in tidal barrages. Studies on the major proposed tidal barrage on the Severn Estuary in England have shown that in the planned basin the minimum water levels would raise enough to permanently flood 80 km2 of intertidal habitats that are essential for the dozens of migratory birds species who stop and nest there. As a result plans to build that barrage have been scrapped.
A series of six tidal lagoons have been proposed instead. They would avoid most of the problems associated with the barrage because they would not impound valuable inter-tidal areas, with the lagoon walls being at their closest about 1.5 km from the coast. At the same time they would extract twice as much energy from the same size of impounded area. As such the tidal lagoon plan has won endorsement from a variety of environmental groups.
The operation of tidal barrages or lagoons does not create greenhouse gases, though their construction does. Greenhouse gas emissions were estimated at 20.5 megatonnes for the construction and operation of the now cancelled Severn Barrage. This may sound like a lot but if this plant was to replace a coal-fired plant then it would pay back all the potential emissions from its construction within 6 months of beginning operation.
Environmental Effects of Tidal Stream Technologies

Meygen
Many studies are being conducted to determine the environmental impact of tidal stream turbines and results so far have been very promising. As the turbines turn much slower than wind turbines, 7-11 RPM for most designs, they move much too slowly to chop up fish or marine mammals as wind turbines do to birds. Research conducted in New York and Maine shows that marine life generally avoids the swinging blades of the turbines anyway, and so far the researchers have found no evidence of damage to fish. Noise from the turbines is another potential issue as marine life can be notoriously sensitive to noise. A study by the U.S. Department of Energy found that young salmon exposed to turbines for “extreme” amounts of time suffered small yet measurable amounts of tissue damage, though the effects lessened as they matured. More study will be required but all in all these were encouraging results.
The placement of arrays of turbines into farms will necessarily slow and reroute currents over a wide area, potentially disrupting fish migration patterns or leading to harmful variations in water pressure. The changes in currents can have an impact upon the ebb and flow of the tides on land, changing their range and impacting inter-tidal ecosystems much like barrages. Irish researchers studying this question found that the impacts could be mitigated enough to make large scale arrays feasible if the turbines were spaced five rotor diameters apart. When gigantic tidal arrays of 8,000 MW were plugged into one study of Scotland’s Pentland Firth researchers found tidal range could be affected by as much as 10% hundreds of kilometres away. On the other hand the farm caused a decrease in turbidity, or sediment in the water, allowing sunlight to penetrate down and trigger phytoplankton blooms which had the effect of boosting the food chain positively from the bottom upwards.
While many of the tests are still preliminary, and scientists have only computer models to go by on the effects of farm arrays, the environmental impacts of tidal stream technologies are generally less than almost all other energy sources.
The carbon emissions from tidal stream technologies derive from the construction, installation and operation of the turbines. In all likelihood the lifecycle carbon emissions from this power source will be comparable to wind and solar.
- Fedorov & Shilin, 2010.
- Clark, 2006.
- Clark, 2006.
- Dadswell & Rulifson, 1994.
- Zhou, Falconer, & Lin, 2014.
- Friends of the Earth Cymru, 2004.
- Fallon, Hartnett & Olbert, 2014.
- Clark, 2006.
Politics of Tidal Power
- The success of wind power indicates the success of tidal power will depend on sustained government investment.

Offshore Wind
On the face of it tidal power would seem to be a total slam dunk from a political perspective. Not only is it carbon-free and renewable, but unlike wind and solar power the tides are entirely predictable and reliable. Furthermore they do not create unsightly views for locals, like wind power, or require large amounts of land like solar, thus neutralizing powerful NIMBY lobbies. Yet the initial hopes placed in tidal barrage power turned sour when France’s pioneering La Rance project inflicted considerable environmental havoc on surrounding marine ecosystems and did not produce enough electricity to justify the construction costs. The only country currently pressing forward with aggressive tidal barrage development schemes is South Korea, though the British government may eventually move forward with tidal lagoon schemes and China with Dynamic Tidal Power.
Tidal stream technologies have only in the past five years begun to mature, and these hold out the prospect of someday bearing bountiful fruit. Governments around the world are sitting up and taking interest and the field is quickly becoming crowded. 19 countries, from Latin America to East Asia, Europe and North America are actively investing in tidal power development. According to a 2014 study by the International Renewable Energy Agency 15 national governments are funding research and demonstration projects, 12 have set up marine testing areas and 11 have imposed feed-in tariffs that subsidize energy to the grid produced by tidal projects. 9 countries have explicit targets for ocean energy generation, the most ambitious of which is France’s goal to have 380 MW of capacity by 2020. Nova Scotia’s 300 MW by 2020 plan is perhaps the world’s most ambitious as France already has 240 MW from the La Rance facility.
Previous experience with wind and solar power shows that it will require sustained and consistent government investment to take these technologies from the drawing board to the demonstration phase and ultimately the commercial utility phase. Once these early steps are taken costs plummet and the companies that developed the early technologies grow to become global giants. Wind generated electricity, for instance, has declined in price over 90% since 1980, making it competitive with most forms of energy production. If the Danish example is anything to go by this robust government investment will be doubly important as Denmark’s early wind power pioneers were working out of garages and small university departments on shoe-string budgets. The unavoidable expenses of designing, placing and testing turbines in an underwater environment means this cottage industry beginning will not be replicable for tidal power. The tidal power companies that will be household names 30 years from now will be those with the kind of economic muscle backing them that can only be provided by governments and large corporations.
Tidal Power Around the World
- Traditional tidal range technologies have largely stalled outside Korea.
- More exotic tidal lagoons and DPS systems are being examined in the UK and China.
- Tidal stream technologies are moving forward rapidly around the world.
Status of Tidal Range Technologies in 2016
The first modern tidal barrage was built in La Rance, France, on the Cherbourg Peninsula in 1966. With a nameplate capacity of 240 MW the facility generates about half a billion kWh annually using 24 low-head Kaplan turbines. Since then only a handful of tidal barrages have been built around the world, including one in Annapolis, Nova Scotia and several small ones in China and Russia.
The only recent major tidal barrage was built at Lake Shiwa in South Korea, which opened in 2011. It has a peak capacity of 254MW and yearly production of 552.7 GWh. The plant was built on the footprint of an old seawall, mitigating much of the environmental damage that has prevented tidal barrage development elsewhere. Currently, the Koreans are looking into the possibility of building and expanding seven more facilities, including the second largest tidal barrage, the Icheron, with potential of 700-1000MW. They are the only country actively pursuing new tidal barrage projects in 2016.
Indicative of the status of tidal barrage technology today is the Severn Barrage which had been proposed for the mouth of the Severn between Bristol and Cardiff. The idea of a tidal power plant on this spot dates to 1925, but justifying the excessive power costs and the environmental damage that would result led the British Government to all but kill the project in 2013. Tidal lagoons have been proposed for the site instead, and the first one is expected to be completed in 2019. For these reasons it seems unlikely much further development of tidal barrages will occur, though tidal lagoons show some promise. The main remaining hope for tidal range technologies remains the gargantuan Dynamic Tidal Power plants proposed for China, though these remain a long way off from being realized.
Status of Tidal Stream Technologies in 2016

Wikipedia
Tidal current turbines have been on the minds of engineers and scientists since the 1970s, but only in the past eight years have they seen sustained investment. As of now the entire sector looks about ready to take off. The British company Marine Current Turbines (MCT) paved the way for tidal projects when it unveiled its SeaGen turbine in 2008. The 1.2 MW tidal energy converter is located in northern Ireland where it provides enough power for about 1,000 homes. Since SeaGen's success, numerous plans for development and pilot projects have emerged, and about 40 different turbine designs are being tested out around the world with the United Kingdom leading the development effort. They are supported by a small club of other countries, with the US, Canada, Norway and the Netherlands having the most active development.
Most of the teams working in these countries were small groups working at universities, with little industrial cohesion and no economies of scale. Nevertheless they have done important pioneering work and the first tidal stream farms are finally being planned. In Scotland construction of the Meygen Project, the world’s first tidal farm, began in early 2015. Meygen will see the carpeting of the seafloor in Pentland Firth with 398 MW worth of 1.5 MW turbines.
In what is perhaps the strongest sign yet that tidal stream technology is reaching a tipping point, the world’s industrial engineering giants are entering the fray, beginning to design their own tidal turbines. Companies like Korea’s Hyundai Heavy Industries, Germany’s Siemens, America’s Lockheed Martin and Japan’s Kawasaki Heavy Industries. If these companies bring their turbine building expertise to bear and dramatically increase the efficiency of the turbines—one Lockheed design has a capacity of 15 MW—then tidal stream technology may start to see the sort of investment needed to catch up with wind and solar.
Tidal Power in Canada
- Nova Scotia and B.C. have the greatest tidal power potential and their industries are discussed in depth in this article.

Gary Fletcher
Because of its geography and innovative entrepreneurs and engineers, Canada is one of the world’s leaders in tidal power. Canada's enormous tidal energy potential exceeds 42 GW, making the country one of the best places for tidal development in the world. There have been 190 suitable sites identified, with BC having the most and Nunavut the greatest total potential. So far most development of tidal power in Canada has been limited to British Columbia and Nova Scotia. The Bay of Fundy, which lies between New Brunswick and Nova Scotia, is Canada's — and possibly the world's — most promising location for tidal power development. Each day, volumes of water in excess of 100 billion tons flow in and out of the bay. That's more than all the world's freshwater rivers combined.
Nova Scotia is betting big on tidal power and has put in place a variety of incentives to encourage research and development of tidal stream technologies and has succeeded in securing foreign investment from a variety of global leaders in the field. The government’s immediate target is for 300 MW of tidal power to be operating in the province by 2020. The nascent tidal power industry may wind up bringing billions in investments and tens of thousands of jobs to Nova Scotia.
British Columbia was also an early leader in tidal stream technology, boasting one of the world’s first demonstration projects at Race Rocks just south of Victoria. Since then however the attitudes of the B.C. and Nova Scotia governments towards the tidal power industries has been a study in contrasts, and British Columbia’s tidal power industry has been left to wither on the vine. This is unfortunate as B.C. has world-class tidal resources and several companies with cutting edge designs that could revolutionize the industry.
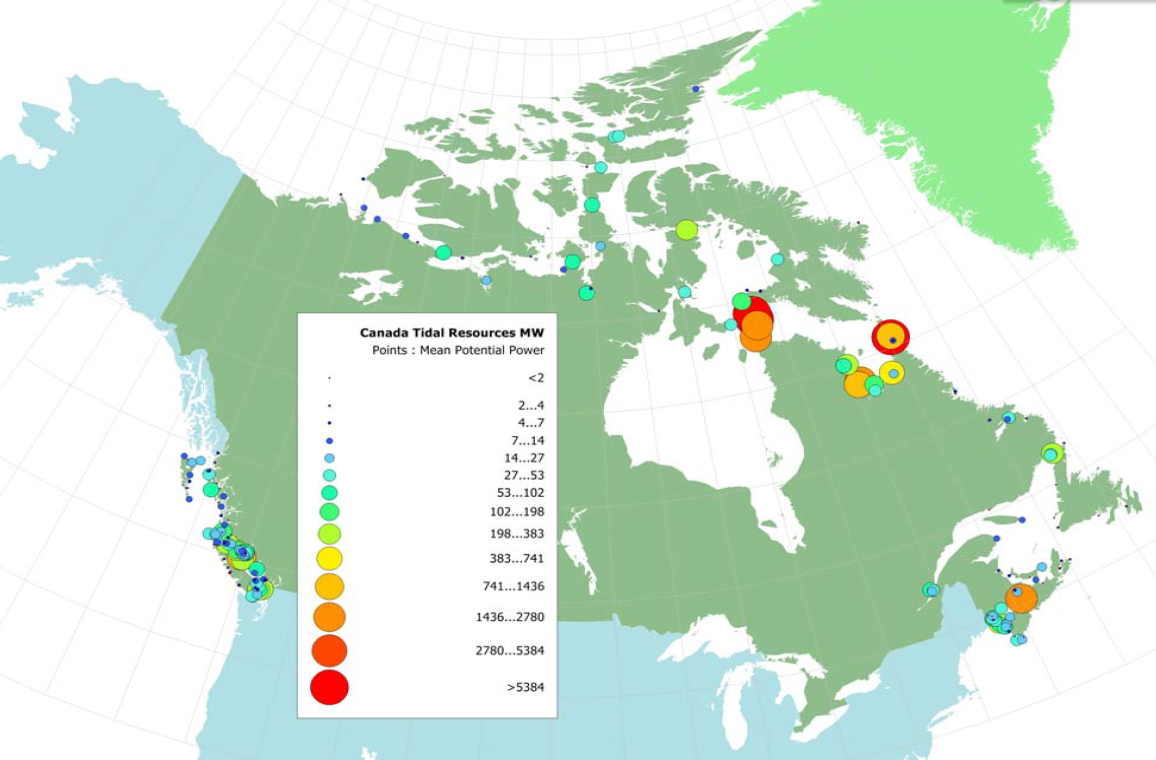
Johnson 2006
Bibliography
To ensure continuity of material, all of the external web pages referenced here were cached in the course of research.
Readers are recommended to search current links for any changes.
American Fisheries Society. "Tidal power development and estuarine and marine environments." Policy Statement. 2010. Cached May 30, 2012.
Aubrecht, Gordon. Energy: Physical, Environmental, and Social Impact. Third Edition. San Francisco, CA: Pearson Education Inc., 2006.
Boronowski, Susan. "Integration of Wave and Tidal Power into the Haida Gwaii Electrical Grid." University of Victoria Department of Mechanical Engineering. 2007. Cached May 30, 2012.
Cameron, Alasdair. "Nova Scotia joins surge on tidal power." Renewable Energy World. 2011. Cached May 30, 2012.
Aquatic Renewable Energy Technologies (AquaRET). "Case Study - Race Rocks." 2006. Cached May 30, 2012.
BC Hydro. "Green Energy Study for British Columbia." Green & Alternative Energy Division. Report No. E44. 2002. Cached May 30, 2012.
Carbon Trust. "Accelerating marine energy." July 2011. Cached April 2, 2016.
Charlier. "Sustainable Co-Generation from the tides: A Review." Renewable and Sustainable Energy Reviews. 2003. Vol 7. Issue 3. Pp 187-213.
Clark, Nigel. "Tidal barrages and birds." British Ornithologists Union, Ibis. Vol: 148 pg. 152-157. 2006. Cached May 30, 2012.
Clark, P, Klossner, R. & Kologe, L. Tidal Energy. Penn State College of Earth and Mineral Sciences. 2003. Cached May 30, 2012.
Clark, Robert. Elements of Tidal-Electric Engineering. London: IEEE Press, 2007.
Colazingari. Marine Natural Resources and Technological Development. New York: Taylor and Francis Group, 2008.
Dadswell, M.J., R.A. Rulifson. "Macrotidal estuaries: a region of collision between migratory marine animals and tidal power development." Biological Journal of the Linnean Society. Vol: 51:1-2. pp 93-113. 1994. Cached May 30, 2012.
Davis, J.K.. "A Review of Information Relating to Fish Passage Through Turbines, Implication to Tidal Power Schemes." Journal of Fish Biology. Vol 33. PP 111-126. 1988. Cached May 30, 2012.
Denny, E. "The economics of tidal power." Power and Energy Society General Meeting. Irish Research Council for the Humanities and Social Sciences. Cached May 30, 2012.
European Innovations Projects. "Annex 6 Potentials for tidal barrages, tidal flows and osmotic power." Retieved April 2, 2016.
Fallon, M. Hartnett, A., & Olbert, S. "The effects of array configuration on the hydro-environmental impacts of tidal turbines." Renewable Energy. Vol. 64 (Apr. 2014): 10-25. ScienceDirect. April 2, 2016.
Fedorov, M. & Shilin, M. "Ecological safety of tidal power projects." Power Technology and Engineering. Vol: 44: 2. pp 22-27. 2010.
Fraenkel. "Next Gen SeaGen." Modern Power Systems. Vol 26. Iss 2. PP 28. 2006. Cached May 30, 2012.
Friends of the Earth Cymru. "A Severn barrage or tidal lagoons?" January 2004. Cached April 2016.
Garrett, C. & Cummins, P. "The power potential of tidal currents in channels." Proceedings of the Royal Society. 2005. Cached May 30, 2012.
Gilbert. "Vancouver Island Project Could Capture Tidal Energy." Journal of Commerce. 2011. Cached May 30, 2012.
Gipe, Paul. "Nova Scotia's proposed ComFIT tariffs circulated." Alliance for Renewable Energy. 2011. Cached May 30, 2012.
Government of Denmark. "Wind Energy Moving Ahead." October 2015. Cached April 2, 2016. P. 20.
Green World Investor. "List of Tidal Power Plants and Future Tidal Stations-Facing Difficult Times." . March 13, 2011. Cached May 30, 2012.
Hammons, T.J. Tidal Power. Proceeding of the IEEE. 1993. Vol 81. Issue 3. PP 419-433.
Halvorsen. "Effects of Tidal Turbine Noise on Fish Hearing and Tissues." U.S Department of Energy. 2011. Cached May 30, 2012.
Harvey. Energy and the New Reality 2: Carbon-Free Energy Supply. Erathscan LTD, 2011. p 313-320.
Ho Bae, Y., Ok Kim, K. & Ho Choi, B. "Lake Sihwa tidal power plant project." Ocean Engineering. 2010. Vol 37: 5-6. p 454-463.
International Renewable Energy Agency. "Tidal Energy Technology Brief." June 2014. Cached April 2, 2016.
Jansen, M. "Severn barrage faces economic rather than environmental hurdles." Ecologist. 2010. Cached May 30, 2012.
Johnson, Jessica. "Tidal energy in Canada." Tidal energy conference. The Ocean Renewable Energy Group. 2006. Cached May 30 2012.
Khan & Bhuyan. "Ocean Energy: Global Technology Development Status." IEA-OES. 2009. Cached May 30, 2012.
Lemperiere, F. & P. Blanc. "Cost-effective large tidal; plants could secure peak power in 15 countries." Hydropower and Dams. 2007. Issue 3. Cached May 30, 2012.
Lena, Manuel. "A sea of electricity." CBS Business Network. 2008. Cached May 30, 2012.
Lee, Kwang-Soo. "Tidal and Tidal Current Power Study in Korea." Coastal Engineering Research Department. Korean Ocean Research and Development Institute. 2006.
Nicholls-Lee, R. & Turnock, S. "Tidal energy extraction: renewable, sustainable and predictable." Science Progress. 2008. 91:1, pp. 81-111.
Marine Renewables Canada. "Marine Renewables Energy in Canada." Cached April 2, 2016.
Martin, Bo. Tidal Power. BC Sustainable Energy Association. 2005. Cached May 30, 2012.
Meygen Limited. "Pentland Firth/Inner Sound." Cached April 2, 2016. www.meygen.com/the-project/pentland-firth-inner-sound/
Ocean Energy Council. "What is Tidal Energy." Cached April 2, 2016.
Pelc & Fujita. "Renewable Energy from the Ocean." Marine Policy. 2002. Vol 26. Issue 6, pp 471-479.
Pollack, John. Both ecology and profit play a role in tidal study. Telegraph Journal. 2008. Cached May 30, 2012.
Pontes & Falcao. "Ocean Energies: Resources and Utilization." Instituto Nacional de Engenharia e Tecnologia Industrial. 2001.
Poyry Management Consulting. "Levelized Costs of Power from Tidal Lagoons." 2014. Cached April 2, 2016.
Renewable Energy World. "Advances in Dynamic Tidal Power Technology." Cached April 2, 2016.
Tidal Energy Today. "Estimate of global potential tidal resources." Cached April 6, 2016. .
Tidaltoday. "Support for Canoe Pass Tidal Energy Consortiums Project." 2009.
Tidal Lagoon Power. "Swansea Bay." Cached April 2, 2016.
Taylor. "Seagen Gets to Go." Alternative Energy. 2008. Cached May 30, 2012.
Westwood, Adam. "Seagen Installation Moves Forward." Renewable Energy Focus. 2008. Vol 9. Iss 3, pp 26-27. Cached May 30, 2012.
Williams. "How France Eclipsed the UK with Brittany Tidal Success Story." Ecologist. 2010. Cached May 30, 2012.
Woolcombe-Adams, C., Watston, M. & Shaw, T. "Severn barrage tidal power project: implications for carbon emissions." Water and Environment Journal. 2008. Vol: 23: 1. pp 63-68. Cached May 30, 2012.